Short Term Test-Retest Reliability of Contralateral Inhibition of Distortion Product Otoacoustic Emissions
Article information
Abstract
Background and Objectives
Strength of medial olivocochlear reflex can be measured reliably using contralateral inhibition of distortion product otoacoustic emissions (DPOAEs) when its fine structure is considered. However, measurement of fine structure of DPOAE is difficult with clinical equipment. Thus, this study investigated the clinically relevant test-retest reliability of contralateral inhibition of DPOAEs.
Subjects and Methods
Twenty-six young adults with normal hearing sensitivity participated. DPOAEs were recorded at 27 discrete f2 frequencies between 800 Hz and 8,000 Hz at frequency resolution of 8 points per octave with and without contralateral white noise presented at 50 dB SPL. To check for short term inter-session reliability, contralateral inhibition of DPOAEs were recorded in three sessions, two recording sessions on first day separated by 30 minutes and third time after one week of the first session. Within each session, DPOAEs were recorded twice in single probe-fit condition to test for intra-session reliability.
Results
Cronbach’s alpha was calculated having poor reliability (α≤ 0.7) of contralateral inhibition of DPOAEs in both intra-session and inter-session conditions for most of the tested frequencies. 95% confidence intervals of contralateral inhibition magnitude also showed large variability.
Conclusions
The current results showed that though DPOAE amplitudes were highly reliable across sessions, amount of inhibition of DPOAEs was not reliable when DPOAEs were measured at discrete frequencies. These findings are concurrent with the literature.
Introduction
When closely spaced two pure tones (f1 and f2, f1<f2) are presented simultaneously, the cochlea generates low level acoustic signals called as distortion product otoacoustic emissions (DPOAEs). DPOAEs are reliable as a clinical tool to monitor cochlear status over time [1]. DPOAEs can be clinically used for: 1) neonatal hearing screening, 2) estimation of hearing status objectively in difficult to test population, 3) differential diagnosis of cochlear and retrocochlear site of hearing loss, 4) monitoring the cochlear status with use of ototoxic drugs, and 5) monitoring the benefits from treatment for sudden sensorineural hearing loss [1]. The DPOAEs are most commonly measured at 2f1-f2 frequency [2]. It comprises of two components in the response, a nonlinear distortion component and linear reflection component [3,4]. When the DPOAEs are measured in ear canal, the constructive and destructive interferences between the two components of DPOAE results in peaks and dips in the response amplitude as a function of frequency. This oscillation in DPOAE phase and level is referred to as DPOAE fine structure [5].
Medial olivocochlear bundles (MOCBs) are efferent pathways in the auditory system which controls functioning of outer hair cells in the cochlea. MOCBs are shown to have a positive effect on various auditory functions in humans, such as speech recognition in noise [6], selective attention [7] and active listening [8]. In addition, studies have also reported that medial olivocochlear (MOC) reflex predicts the success achieved from auditory perceptual training [9] and can be used to measure the success obtained with auditory perceptual training [10]. Clinically, MOCB activity can be elicited non-invasively by means of acoustic stimulation either ipsilaterally, contralaterally or bilaterally. The acoustic stimulation typically results in reduction in the amplitude of otoacoustic emissions (OAEs), referred as inhibition of OAEs. Acoustic stimulation to the contralateral ear, termed as contralateral inhibition of OAE has been widely used to measure the functioning of the efferent pathways among humans. Many of the researchers have measured MOCB activity using contralateral inhibition of click evoked or transient evoked otoacoustic emissions (TEOAEs) [6-8,10] and contralateral acoustic stimulation (CAS) has shown to reduce the amplitude of transient evoked otoacoustic emissions for various types of noise [11]. The effects of CAS on the DPOAE has been reported by several investigators [5,12-15] and findings of these investigations have reported both reduction and enhancement of amplitude of DPOAEs [12,13,16,17].
MOC reflex has been successfully used in several clinical applications. The clinical applications include, to measure the effectiveness of auditory training [9,10], to differentiate individuals with auditory neuropathy spectrum disorder [18] and auditory processing disorders [19] from normal individuals. To ensure that the MOC reflex can be reliably used in those clinical applications, the knowledge on its test-retest stability and reliability is important.
A study has shown that contralateral inhibition of TEOAEs is a reliable measure [20]. These investigators reported good short-term reliability (α≥0.8) of contralateral inhibition of TEOAEs between 1 and 4 days for most of the test conditions. Similarly, the short-term stability of MOC reflex using DPOAEs has been investigated by few investigators [5,15,21]. Wagner, et al. [21] repeated the measurement of contralateral inhibition of DPOAEs using custom made research equipment on average of 5.5 days. In one of their protocols, the effect of CAS was measured at the dip of DPOAE fine structure. Wagner, et al. [21] reported a repeatability coefficient of 1.21 dB and this value was exceeded in only 5% of their participants, thus indicating a good short-term test-retest repeatability. Kumar, et al. [15] assessed the test-retest reliability of the magnitude of contralateral inhibition of DPOAEs using commercially available OAE equipment over nine different sessions within a short period of 15 days. The DPOAEs were recorded with f2/f1 ratio of 1.20 for seven pairs of frequencies with f2 at 1,000, 2,000, 3,000, 4,000, 5,000, 6,000, and 8,000 Hz. They observed that the intra-session reliability ranged between 0.2 and 0.7 (Cronbach’s alpha) whereas the inter-session repeatability of contralateral inhibition of DPOAE magnitudes ranged between 0.5 and 0.8 depending on the frequency. Thus, the DPOAE inhibition magnitude was having poor reliability at most of the frequencies tested. One of the reasons proposed by the authors for poor reliability of contralateral inhibition of DPOAEs was that the study did not consider DPOAE fine structure. Overall poor reliability results with commercially available OAE equipment prompted Kumar, et al. [15] to discourage the use of contralateral inhibition of DPOAEs for the clinical measurement of MOC reflex. Mishra, et al. [5] aimed to assess the short-term (tested on four consecutive days) and long-term (tested weekly for four weeks) reliability of MOC reflex with fine structure DPOAEs using custom made OAE measurement and analysis software. They tested four different MOC reflex indices to see whether any one measure was more stable than another. All the measurements were done at the maxima of the fine structure DPOAEs using custom made research equipment. Measurement of MOC reflex at the peak showed that 96% of the data points of the short-term reliability and 90% of the data points of long term reliability had inhibition and not enhancement. Cronbach’s alpha ranged between a low of 0.57 and a high of 0.97 while the long term reliability coefficient ranged between 0.63 and 0.97 for various indices that they measured. Based on these results, Mishra, et al. [5] concluded that the ßMOC reflex measured with DPOAEs are stable over time. However, these authors rightly pointed that the study should be validated with a larger group in clinical conditions before generalization can be made to clinical populations.
From the current literatures, it is clear that the effect of CAS on DPOAE is reliable in short-term as well as long-term with custom made measurement and analysis software when its fine structure is taken into consideration. However, with commercially available OAE equipment, measurement of fine structure DPOAEs may not be clinically feasible, thus leading to less reliable effect of CAS on DPOAEs. Measurement of fine structure DPOAE is time consuming and will not be available in most of the clinically used instruments for the measurement of DPOAEs. Hence, measurement of MOC reflex using DPOAE measured at discrete frequencies can be a viable option in terms of availability of equipment and time consumption, but less reliable. Therefore, measurement of DPOAEs at discrete frequencies to observe MOC reflex may not be a good choice. Thus, there is a need to identify a frequency resolution to measure MOC reflex using DPOAE which is less time consuming, reliable, as well as feasible with the commercially available clinical OAE equipment. Therefore, the present study aimed to measure the short-term test-retest reliability of contralateral inhibition of DPOAEs using a clinical OAE instrument at large number of discrete f2 frequencies. Specifically, the objective of the study was to measure the stability of MOC reflex using DPOAEs measured at 8 points per octave over a period of one week. This frequency resolution was thought to be a balance between time consuming fine structure DPOAE and less reliable low resolution discrete frequency DPOAE. Most of the studies measuring short-term reliability have used duration between five to ten days and hence one-week duration was considered to measure short-term reliability in this research [1,5,22].
Subjects and Methods
Participants
A total of 26 participants (10 males, 16 females) aged between 18 and 25 years participated in the study. All the participants had air conduction hearing thresholds less than 15 dB HL in both ears at octave frequencies between 250-8,000 Hz. None of them had history of otologic or neurologic problems and exposure to ototoxic medications or hazardous noise. Before the data collection, immittance examination was performed and its result showed A-type tympanogram [23] with acoustic reflex thresholds at normal levels for all the participants. The acoustic reflex threshold for broadband noise was also measured and it was found to be greater than 75 dB SPL for all participants. All procedures used in the study adhered to the institutional ethical committee guidelines (IEC KMC MLR 11-13/217) and followed the principles of the Declaration of Helsinki. All participants were explained about the purpose of the study and informed consent was obtained.
DPOAE recordings
During the recording of DPOAEs, participants were made to sit on a reclining chair in a comfortable position and probe was placed in the right ear of participants. Participants were instructed to remain calm and not to move their head throughout the recording session. DPOAE was recorded using ILO v6 (Otodynamics Ltd., Hatfield, UK) otoacoustic emission system. DPOAE was recorded at f2 frequencies ranging between 800 and 8,000 Hz at a frequency resolution of 8 points per octave, and the f2/f1 ratio was kept constant at 1.20. The effect of CAS on DPOAE was measured by recording DPOAE in the presence of broadband noise in the contralateral ear. The broadband noise was generated using GSI-61 clinical audiometer (Grason-Stadler Inc., Madison, WI, USA) and delivered to the contralateral ear of participants using ER-3A insert phones (Etymotic Research, Inc., Elk Grove Village, IL, USA) at 50 dB SPL. The level of noise was calibrated using 2 cc coupler. All the recordings of DPOAEs were carried out in a sound-treated room.
The contralateral inhibition of DPOAE was measured in multiple sessions to check for inter-session and intra-session reliability. On the first day, DPOAEs was recorded in two sessions separated by 30 minutes, referred to as first and second recording sessions respectively. The third recording was carried out after a gap of one week, referred as third recording session. In each session, four recordings of DPOAE was obtained, two recordings without CAS (quiet) and two recordings with CAS. All the recordings within a session was completed without disturbing the placement of OAE probe. Comparison of inhibition of DPOAEs within session provides a measure of intra-session reliability and comparison of inhibition of DPOAEs across sessions provides a measure of inter-session reliability of contralateral inhibition of DPOAEs. The timeline of DPOAE recordings is shown in Fig. 1. All the 26 participants completed two sessions of DPOAE, while the third session was completed in 16 participants.

Timeline of DPOAE recordings in this study. DPQ1: first recording of DPOAE without CAS, DPN1: first recoding of DPOAE with CAS, DPQ 2: second recoding of DPOAE without CAS, DPN2: second recording of DPOAE with CAS. DPOAE: distortion product otoacoustic emissions, CAS: contralateral acoustic stimulation.
Data analysis
DPOAE was considered to be present if the signal-to-noise ratio (SNR) was greater than 6 dB. The criteria of 6 dB SNR has been commonly used in most of the studies [5,15,21]. The mean amplitude of DPOAEs were computed separately for recordings obtained without CAS (Session 1: DPQ1 & DPQ2; Session2: DPQ1 & DPQ2; Session 3: DPQ1 & DPQ2) and with CAS (Session 1: DPN1 & DPN2; Session 2: DPN1 & DPN2; Session 3: DPN1 & DPN2) conditions at each test frequency. The amount of contralateral inhibition of DPOAEs was calculated by measuring the difference in the amplitude of DPOAE with and without CAS. A total of six DPOAE inhibition values were obtained from three sessions (Session 1: IN1 & IN2; Session 2: IN3 & IN4; Session 3: IN5 & IN6). Test-retest reliability can be analyzed in several ways including the estimation through Cronbach’s alpha, intraclass correlation coefficient, or comparing the mean differences through the t-test. Cronbach’s alpha was chosen because, most of the studies which investigated the test-retest reliability of DPOAE inhibition have used this measure, thus making the comparison of this study with the earlier studies easier. Cronbach’s alpha and 95% confidence interval was calculated for the magnitude of inhibition of DPOAEs and the amplitude of DPOAEs to assess intra-session and inter-session reliability of inhibition of DPOAEs and amplitude of DPOAEs. The magnitude of inhibition of DPOAEs across recordings within each session was compared to check for intra-session reliability (IN1-IN2). Inter-session reliability was measured by comparing the magnitude of inhibition of DPOAEs across the sessions (IN1-IN3-IN4-IN5-IN6). All statistical analysis was done using Statistical Package for the Social Sciences (SPSS, version 17, SPSS Inc., Chicago, IL, USA).
Results
In the present study, DPOAEs could be obtained from all the participants across the sessions. Fig. 2A shows the mean amplitude of DPOAEs without CAS across the sessions. From Fig. 2A, it can be seen that the mean amplitude of DPOAEs without CAS did not vary much across the sessions. Fig. 2B shows the mean difference in the magnitude of inhibition of DPOAEs in single probe fit condition (intra-session) and multiple probe fit conditions (inter-session). The mean intrasession DPOAE amplitude difference ranged between 0 dB SPL and 0.9 dB SPL while the mean inter-session DPOAE amplitude difference ranged between 0 dB SPL to 1.2 dB SPL. Thus, the inter-session DPOAE amplitude difference range was slightly higher than the intra-session DPOAE amplitude variation. Shapiro-Wilk test revealed that the DPOAE amplitudes were normally distributed. Cronbach’s alpha was calculated at each frequency to check for the reliability of DPOAE amplitudes. Results showed that the Cronbach’s alpha values ranged between 0.94 and 0.994 across frequencies which point toward excellent reliability [24]. This finding indicates that the amplitude of DPOAEs are similar or highly reliable over a short time period.
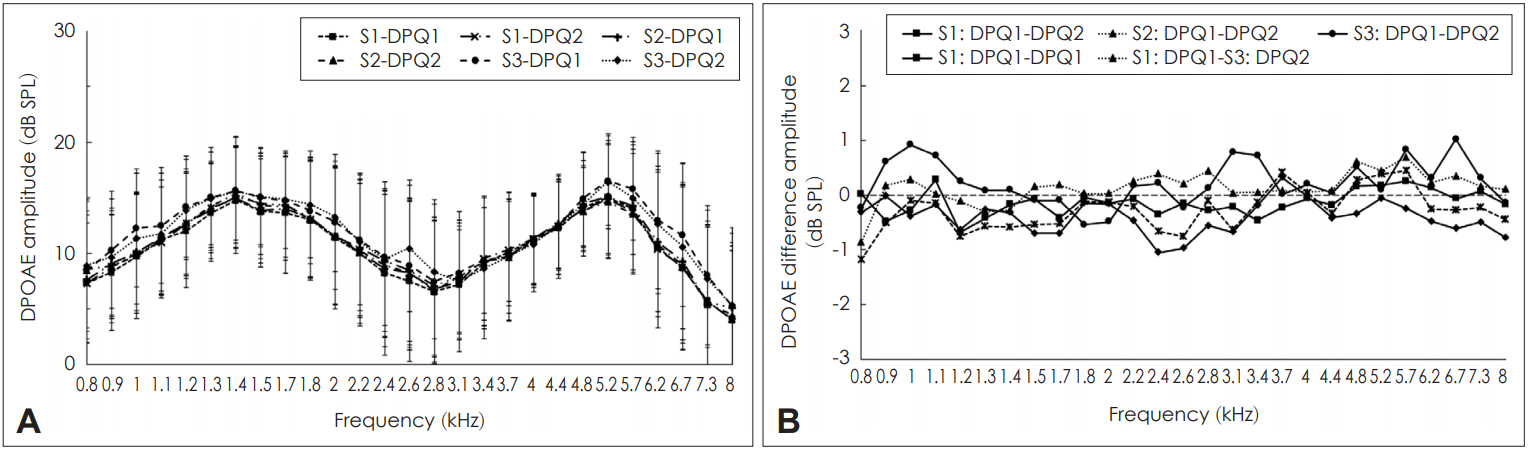
Variability of distortion product otoacoustic emission (DPOAE) amplitude across sessions. A: Mean amplitude of DPOAEs (along with one standard deviation) without contralateral acoustic stimulation (CAS) for various frequencies across the sessions. B: Mean difference in intra-session and inter-session DPOAE amplitude in three sessions. S1-DPQ1: amplitude of DPOAE without CAS in first recording of first session, S1-DPQ2: amplitude of DPOAE without CAS in the second recording of first session, S2-DPQ1: amplitude of DPOAE without CAS in the first recording of second session, S2-DPQ2: amplitude of DPOAE without CAS in the second recording of second session, S3-DPQ1: amplitude of DPOAE without CAS in the first recording of third session, S3-DPQ2: amplitude of DPOAE without CAS in the second recording of third session.
White noise in the contralateral ear resulted in either inhibition or enhancement of DPOAEs across the frequencies in all the participants. Occasionally at few frequencies in few participants, lack of inhibition was also noticed. Inhibition of DPOAEs across sessions are shown in Fig. 3. Fig 3A showed that the magnitude of inhibition of DPOAEs varied across frequencies and across sessions. The difference in the magnitude of inhibition across sessions are shown in Fig. 3B. It showed large variations in the magnitude of inhibition of DPOAEs both within and across sessions. Within and across session variations were frequency dependent with few frequencies having less variation and few frequencies having more variation. To assess the intra-session and inter-session reliability of inhibition of DPOAE, Cronbach’s alpha was calculated separately for each condition. The results are shown in Table 1. In addition, 95% confidence intervals for the difference were also calculated whose results are also shown in Table 1. Table 1 reveals that among the 27 frequencies tested, the intra-session reliability of inhibition of DPOAE was unacceptable (α<0.5) at 16 frequencies. Questionable reliability (0.5≤α<0.7) was obtained for seven frequencies, acceptable reliability (0.7≤α<0.8) and good reliability (0.8≤α<0.9) was obtained at two frequencies each. None of the frequencies lower than 2,380 Hz had acceptable reliability. Two frequencies beyond 6,726 Hz also showed less than acceptable reliability. Thus, based on the findings of present study, it could be believed that the intra-session reliability for inhibition of DPOAEs are reliable at mid and high frequencies. The 95% confidence intervals for both within session and across sessions showed a large range and greater variability. This compliments the results of Cronbach’s alpha reliability values.
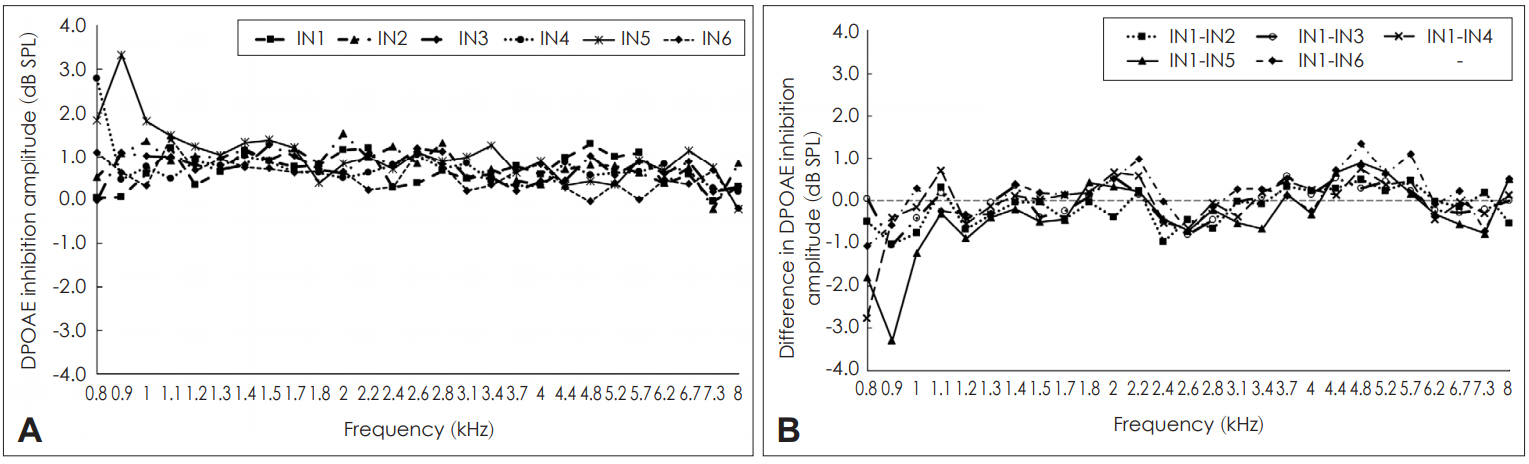
Inhibition of distortion product otoacoustic emission (DPOAE) amplitude across sessions. A: Mean inhibition of DPOAE amplitude across different sessions. B: Mean difference in the amount of inhibition of DPOAE amplitude within (intra) and across (inter) sessions. IN1: mean inhibition of DPOAE amplitude in first recording of first session, IN2: mean inhibition of DPOAE amplitude in second recording of first session, IN3: mean inhibition of DPOAE amplitude in first recording of second session, IN4: mean inhibition of DPOAE amplitude in second recording of second session, IN5: mean inhibition of DPOAE amplitude in first recoding of third session, IN6: mean inhibition of DPOAE amplitude in second recording of third session.

Intra-session and inter-session Cronbach’s alpha for contralateral inhibition amplitude of distortion product otoacoustic emissions along with 95% confidence intervals
The Cronbach’s alpha for inter-session reliability of inhibition of DPOAE was unacceptable for 19 frequencies, questionable for six frequencies, and good for two frequencies. The frequencies which had reliable inter-session DPOAE inhibition were scattered across the frequencies tested. In addition, when the frequencies at which acceptable reliability was obtained for intra-session and inter-session were compared, only four frequencies were in common (2,380, 2,832, 5,188, and 6,726 Hz). Similar to intra-session reliability, inter-session reliability for inhibition of DPOAEs also showed greater variability as indicated by large 95% confidence intervals. Thus, the results of the present study show that though the amplitude of DPOAE was reliable, short-term test-retest reliability for inhibition of DPOAE was poor.
Discussion
The present study investigated short-term test-retest reliability of inhibition of DPOAEs by recording DPOAEs at higher resolution of eight points per octave. In addition to reliability of inhibition of DPOAEs, reliability of DPOAE amplitude was also measured. Findings of the present study showed high test-retest repeatability for the amplitude of DPOAEs with Cronbach’s alpha values greater than 0.9 at all the measured frequencies. This finding was comparable with the literature which also reports high test-retest reliability for DPOAE amplitudes [1,15]. Thus, suggesting that the amplitude of DPOAEs were similar across the sessions and highly reliable. Results also showed that the variations in the amplitude of DPOAE was variable across frequencies in both intra-session and intersession conditions. This observation was also consistent with the findings of Kumar, et al. [15] who observed different amount of mean difference across frequencies. However, findings of the present study as well as Kumar, et al. [15] did not reveal any identifiable trend across frequencies. Thus suggesting that slight variation across test frequencies is expected during repeated measurements. On the other hand, test-retest reliability for inhibition of DPOAE was poor in the present study consistent with the findings of earlier investigation [15]. This poor reliability is a consequence of large confidence intervals for magnitude of inhibition of DPOAEs. Large confidence interval for magnitude of inhibition of DPOAEs has been reported by previous studies [5,15].
Intra-session reliability of DPOAE inhibition was greater than 0.5 for frequencies between 2,380 Hz and 6,726 Hz. This finding in the present investigation suggests that inhibition of DPOAEs are reliable when recorded at mid and high frequencies. Mishra, et al. [5] observed slightly poorer reliability when the DPOAE maxima was ranging between 1,450 Hz and 1,600 Hz. Variations in intra-session reliability for inhibition of DPOAEs across frequencies was also observed by Kumar, et al. [15]. Comparison of findings across investigations does not agree on any particular frequency or range of frequencies that could be used to measure inhibition of DPOAEs reliably.
Comparison of magnitude of reliability across frequencies between intra-session and inter-session conditions showed that the Cronbach’s alpha was greater than 0.5 only for four frequencies. These results in the present investigation are in agreement with the findings of Kumar, et al. [15] where intra-session reliability coefficients ranged between 0.2 and 0.7 and inter-session reliability coefficients between 0.5 to 0.8. Their results also showed poor reliability of contralateral inhibition of DPOAEs at majority of the frequencies. On the other hand, results of the present study contradicts the findings of Mishra, et al. [5] who reported high intra-session and intersession reliability for the inhibition of DPOAEs when measured at peaks of DPOAE fine structure.
Several factors such as ambient noise levels, changes in the hearing status, changes in middle ear status, multiple experimenters could affect test-retest reliability of DPOAE inhibition. In the present investigation care was taken to eliminate the effect of these factors. All the recordings were carried out in a controlled environment, in an acoustically treated room which will result in minimal variations in ambient noise. Moreover, variation in ambient noise, if any, should also have resulted in variations of the absolute amplitude of DPOAEs, which was not the case. Therefore, it was unlikely that variability in the magnitude of inhibition was due to ambient noise levels. Another possible reason for poor reliability could be the probable changes in the hearing status and middle ear status which could affect the amplitude of DPOAEs which in turn affects the inhibition of DPOAEs. However, any changes in the hearing status or middle ear status would also have changed the amplitude of DPOAE, which in fact has remained stable throughout the study period. Therefore, influence of any physiological changes in the participants’ auditory system can be ruled out as the probable factor for present results. Same experimenter did the testing on all the participants and same probe was used for one participant during multiple recordings. Hence, these variables can be excluded as the probable reason for poor reliability.
Apart from the above mentioned variables, other possible reasons for poor reliability of DPOAE inhibition magnitude which were not controlled in this study. Effect of CAS on DPOAEs were measured in passive listening condition and the attention of the participants towards the stimuli was not controlled. There are possibilities that few participants might have attended the stimuli and others did not pay attention which can result in different amounts of DPOAE inhibition during CAS. Furthermore, within the same participant also attention might have varied across different sessions which can result in variations in the magnitude of DPOAE inhibition. Another major factor that could have caused the poor reliability of the magnitude of DPOAE inhibition is the measurement of DPOAEs at discrete f2 frequencies. In the present study, we used a frequency resolution of eight points per octave which was greater than that used by Kumar, et al. [15], who measured DPOAE inhibition at seven discrete frequencies. However, the frequency resolution used in the present study is not sufficient to measure the fine structure of DPOAEs which consists of peaks and dips. In addition, literature reports that generally, the effect of CAS on DPOAE is inhibitory in nature at fine structure peaks, while it is enhancing in nature at fine structure dips [5]. Also, the peaks and dips of the fine structure is peculiar to an individual participant, that is, the frequencies at which peaks and dips occur for each individual would be different. Thus, when DPOAEs are measured at specific fixed frequencies as done in this research, it is not possible to understand whether a measurement has been done at a peak frequency or at a dip frequency. Hence, it is highly possible that we could have measured the effect of CAS on DPOAEs at the peak frequency for few participants while it could be at dip frequency for few others. Furthermore, while averaging the effect of CAS on DPOAEs from all the participants, both inhibitions as well as enhancements are included which will reduce the mean effect of contralateral noise [5], thereby reducing the reliability of inhibition magnitude of DPOAEs. Therefore, it appears that the major reason for poor repeatability of contralateral inhibition of DPOAEs observed in the present study is due to lower frequency resolution of DPOAE measurement at fixed frequencies. This suggests that the inhibition of DPOAE measured at discrete frequencies may not be reliable, especially across different sessions in a clinical set up.
Clinically, to measure the effect of CAS on DPOAE at its fine structure peak or dip using a lesser frequency resolution, a guideline was suggested by Deeter, et al. [13]. These researchers observed that the periodicity of fine structure in humans is estimated to the value of f/delta f=16 where, f is the center frequency and delta f is the frequency distance between adjacent maxima or minima. With this estimation, Deeter, et al. [13] speculate that measurements of inhibition of DPOAE can be done at five frequencies: f, f±(f/4), and f±(f/8), where f is the frequency of interest. Such a selection of frequency would make the DPOAE measurement at or near the peak of the fine structure period, thereby making it possible to observe the effect of CAS on DPOAE peak at least at that frequency region. This is a probable solution for a clinician who cannot measure fine structure DPOAE with a clinical equipment. However, this would be possible only if the clinical equipment provides the option of specific individual frequency selection. This method was not implemented in the current research and its clinical application can be clinically validated in future studies.
It is suggested that MOC reflex measured using fine structure DPOAE is stable over time and can also be used to detect the difference between groups with normal hearing and groups with auditory impairment [5]. Especially, measurement of MOC reflex at the fine structure peak is said to be more reliable than at dip [5]. These authors also suggested that fine structure DPOAE based measures can be possibly used to monitor intervention-related success. Generally, clinical DPOAE equipment will have a maximum frequency resolution between eight and twelve points per octave with fixed frequencies. Our study investigated the test-retest reliability of contralateral inhibition of DPOAEs using clinical OAE equipment. Effect of CAS on DPOAEs were measured between 800 Hz and 8,000 Hz with a frequency resolution of eight points per octave. Result showed that the magnitude of contralateral inhibition of DPOAE is variable in both within and across sessions even though the amplitude of DPOAE remained stable across the recordings. Probable reasons for this poor reliability in this research has been discussed above. Current results suggest that measurement of the effect of CAS on DPOAEs using a lower frequency resolution with fixed frequencies may not provide reliable results. This might hinder a clinician from obtaining the benefit of fine structure based DPOAE measurements of MOC reflex which is stable. However, measurement of inhibition of DPOAE at specific frequencies at or near the estimated fine structure peak might provide reliable result with a clinical equipment, which has to be explored in future studies.
Notes
Conflicts of interest: The authors have no financial conflicts of interest.